UV Optics: Tighter Tolerances and Different Materials
The UV Challenge – Tighter Surface Specifications
The main challenge associated with manufacturing ultraviolet (UV) optics is that surface tolerances must be much tighter than those of visible and IR optics. The standard P-V irregularity tolerance for precision lenses is λ/10, therefore the physical accuracy required in manufacturing is dependent on the wavelength at which the optic will used. Because tolerances are quoted at a fixed wavelength of 632.8nm, λ/10 implies 63.28nm regardless of design wavelength.
Relative performance will be worse at short UV wavelengths. For instance, a lens used at 308nm will require an irregularity tolerance twice as tight as a lens used at 632.8nm to maintain the same relative level of wavefront distortion. This same principle also applies to optical coatings. The thickness of simple coatings are typically specified to quarter or half wavelengths of light. For UV coatings, the deposition process requires much more accurate monitoring; small fluctuations in production results in much greater errors in the UV than they would in the visible or IR spectra.
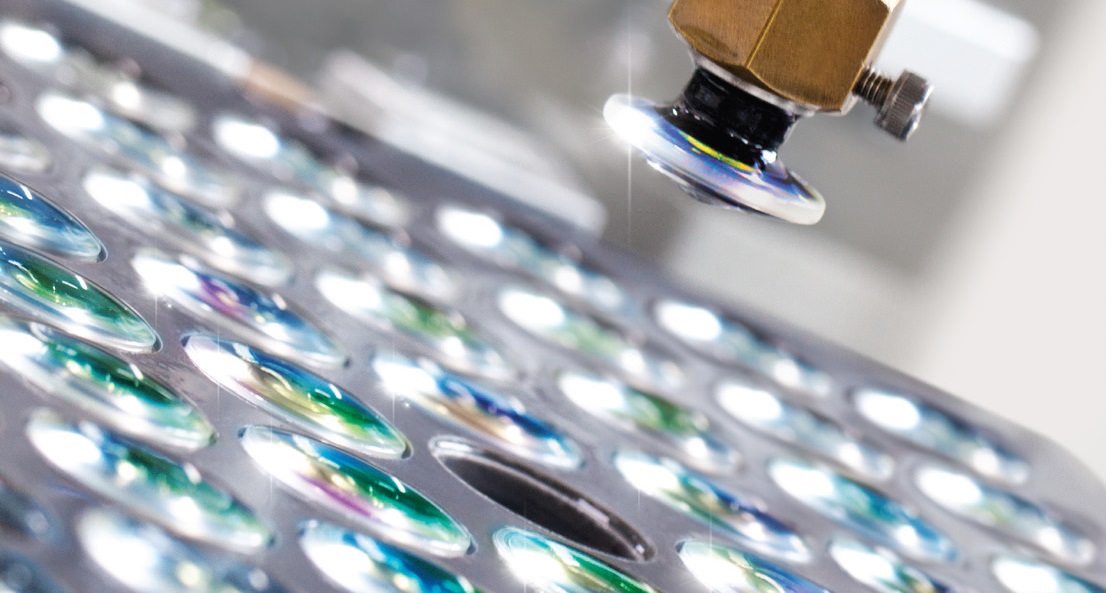
Figure 1: Tighter surface specifications are required when manufacturing UV Optics
The short wavelengths of UV light typically absorb and scatter much more than visible or IR light. Surface imperfections such as scratches and pits are amplified under UV light and even the smallest surface flaws can be points of absorption or scatter, reducing system throughput. In order to minimize energy loss, a tight surface quality specification is required. While the standard surface quality for precision lenses used with visible light is 40-20, a surface quality of 10-5 may be required for UV applications. Scattering can lead to energy loss, a reduction in the efficiency of your optical system, and even false signals in applications using imaging sensors or other detectors. UV materials exhibit greater dispersion than visible or IR materials, leading to significant aberration in broadband UV applications. To avoid this, many UV optical systems use reflective optics to avoid dispersion inside the bulk material.
Optical Substrates and Coatings
Absorption and scatter not only lead to a loss in throughput, but can also result in component damage and failure. Too much UV absorption may actually bleach a substrate and alter its chemical properties, leading to component failure. In order to prevent this, UV substrates must fully transmit the entire desired wavelength range and be polished to near perfection. Some of the most common UV transmitting substrates include UV Fused Silica, Calcium Fluoride (CaF2), and Sapphire. UV Fused Silica is the most commonly used UV substrate due to its affordability, accessibility, and easy fabrication compared to many other UV-transmitting materials. UV Fused Silica also transmits wavelengths down to 193nm and offers a low coefficient of thermal expansion. CaF2 has a low refractive index, low axial and radial birefringence, and transmits wavelengths down to 180nm, making it suitable for UV excimer laser applications. Sapphire is ideal for use in harsh environments because of its extreme surface hardness, high thermal conductivity, high dielectric constant, and strong resistance to a variety of chemical acids or alkalis. With extreme resistance to UV darkening, high quality sapphire is often used in high power UV applications and some types of optical Sapphire transmits down to 150nm. Birefringence is one disadvantage of sapphire, but when cut properly along the crystal’s C-axis, birefringence is minimized.
UV absorption can also bleach and damage optical coatings, not just the bulk material. Because of this, different coating materials are needed for both transmissive and reflective optics operating within the UV spectrum. Deep-UV (DUV) mirror coatings are also particularly sensitive to small coating thickness errors because material limitations in the UV produce relatively narrowband reflectors.
Multiphoton Absorption
Light only transmits through transmissive optics without being absorbed when it has an energy smaller than the substrate’s bandgap energy and cannot excite electrons from the valence band of the material to the conduction band. Multiphoton absorption occurs when two or more photons are spontaneously absorbed to excite an electron into the conduction band, causing light that would normally transmit through the optic to be absorbed. UV light has more energy than visible and IR radiation because the energy of light is inversely proportional to wavelength. Multiphoton absorption can become substantial in the UV and at high optical intensities, increasing absorption and starting a runaway process which will ultimately damage the optic. Single-photon absorption is linearly dependent on the intensity of incident light, but multiphoton absorption is dependent on the square of the light intensity and will dominate over linear absorption at high intensities. Materials with a high refractive index are especially susceptible to multiphoton absorption because they have a small band gap energy.
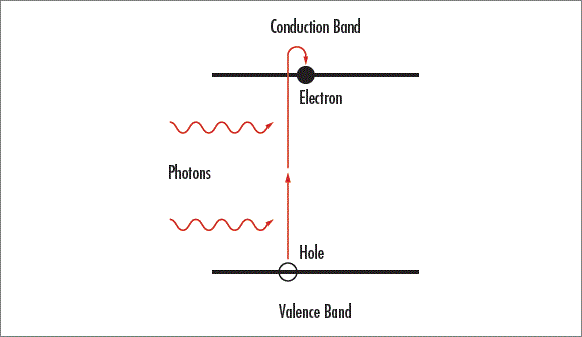
or view regional numbers
QUOTE TOOL
enter stock numbers to begin
Copyright 2023, Edmund Optics Inc., 14F., No.83, Sec. 4, Wenxin Road, Beitun District , Taichung City 406, Taiwan (R.O.C.)
California Consumer Privacy Act (CCPA): Do Not Sell My Information